The MolMatTC group accumulates a great experience in the application of the state-of-the-art quantum chemistry methods for the characterization of electroactive molecular systems. By means of quantum-chemical calculations, we try to establish relationships between the molecular structure of a system and its electronic, redox, and optical properties. An in-depth knowledge of such relationships is required in order to design novel electronic and optoelectronic devices with improved features.
Currently, the MolMatTC group is mainly focused on four different research lines:
-
Functional supramolecular systems
-
Exciton/Charge transport in (supra)molecular materials
-
Hole and electron transporting materials for perovskites solar cells
-
Ionic transition-metal complexes for electroluminescent devices
Functional supramolecular systems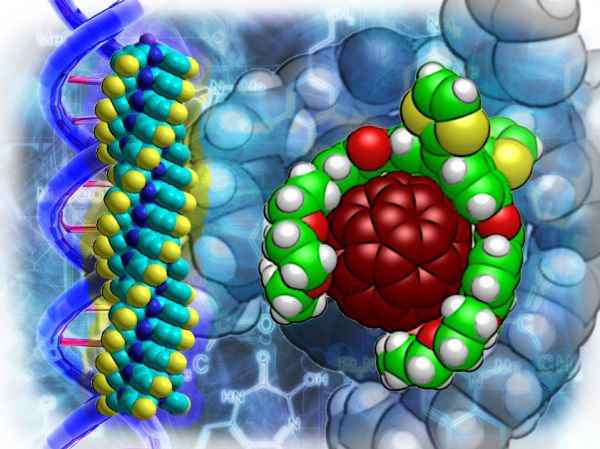
We are interested in the study of supramolecular systems governed by noncovalent interactions. These weak but important noncovalent forces are responsible for the organization of the molecular aggregate and, therefore, for the functional properties (chirality, optical properties, exciton/charge transport, etc). In the research group, we are focused on the characterization of two different supramolecular arrangements:
1) Donor-acceptor (D-A) supramolecular complexes involving carbon nanoforms as the electron active acceptor systems. These D-A supramolecular systems can give rise to an efficient photoinduced charge-transfer process which is crucial for photovoltaic applications. These studies are done in close collaboration with Prof. Nazario Martín (Univ. Complutense Madrid) and Dr. Emilio M. Pérez (IMDEA-Nanoscience, Madrid).
2) Supramolecular polymers based on C3-symmety π-conjugated compounds endowed with amide functional groups that promote helical aggregates with interesting chiral and optical properties. These studies are done in close collaboration with Prof. Tomás Torres (Univ. Autónoma de Madrid), Dr. David González (Univ. Autónoma de Madrid) and Dr. Luis Sánchez (Univ. Complutense Madrid).
From a theoretical perspective, the description of noncovalent interactions represents a great theoretical challenge for most quantum chemistry methods. High-level ab initio methods able to accurately deal with the electron-correlation phenomenon and, thus, can offer a proper description of these subtle forces. However, these methods can only be applied in systems of small molecular size. In last decade, great efforts have been done to develop practical methodologies to tackle the problem of the noncovalent interaction in the context of the density functional theory. In this sense, the group has been actively worked in the last 5 years in the development and applications of novel approximations coupled with modern density functional to be able to describe supramolecular systems in a balance way.
Selected publications:
1) M. Gallego, J. Calbo, J. Aragó, R. M. Krick Calderon, F. H. Liquido, T. Iwamoto, A. K. Greene, E. A. Jackson, E. M. Pérez, E. Ortí, D. M. Guldi, L. T. Scott, N. Martín, Angew. Chem. Int. Ed. 2014, 53, 2170.
2) J. Guilleme, M. J. Mayoral, J. Calbo, J. Aragó, P. M. Viruela, E. Ortí, T. Torres, D. González-Rodríguez, Angew. Chem. Int. Ed. 2015, 54, 2543-2547
3) J. Calbo, E. Ortí, J.C. Sancho-Garcia and J. Aragó J. Chem. Theory Comput. 2015, 11, 932-939
Exciton/charge transport in (supra)molecular materials
Exciton and charge transport in molecular materials are two fundamental physical processes taking place in many organic electronic devices including light emitting diodes and solar cells. For example, a long exciton diffusion length is a prerequisite for efficient organic solar cells, where excitons should diffuse to a donor-acceptor interface to initiate the process of charge separation. Subsequently, the holes and electrons generated in the donor and acceptor material need to efficiently migrate to the corresponding electrodes for the generation of photocurrent. Consequently, high charge-carrier mobilities in the organic semiconducting materials are also necessary for an optimal performance of the (opto)electronic devices.
Our current interest is in studying the exciton transport in supramolecular aggregates; in particular, in the helical systems (see the previous research line) in which we have accumulated a wide experience in the theoretical description by using quantum (DFT) and classical (molecular dynamics) methods. In addition to the quantum and classical calculations, we design model Hamiltonians to describe the exciton transport in complex molecular aggregates in a simplified way.
We have also interested in the theoretical description of the photoinduced electron transfer processes in D-A supramolecular complexes based on carbon nanoforms by using proper rate expressions where the most relevant transport parameters included in the rate expression can be derived from first-principles electronic structure calculations.
Selected publications:
1) J. Aragó and A. Troisi Phys. Rev. Lett. 2015, 114, 026402
2) J. Aragó and A. Troisi Adv. Funct. Mater. 2016, 26, 2316-2325
Hole and electron transporting materials for perovskites solar cells

In the search for low-cost alternatives to power sources based on fossil fuels, organic-inorganic hybrid perovskite solar cells have emerged as a potentially disruptive technology. Intensive research efforts have boosted the power conversion efficiencies (PCE) in photovoltaic (PV) devices based on these materials from around 4% to a certified 22.1% in the last three years. Because they are more compatible with multi-junction architectures (e.g. to be combined as top cells in existing silicon technology), interest has shifted away from mesostructured cells (where the perovskite infiltrates a mesoporous scaffold) to (the also simpler) planar heterojunctions, where the perovskite is sandwiched between hole- and electron-transporting material (HTM and ETM) contacts. In contrast to organic photovoltaic cells where a significant energy offset between electron donors and acceptors is required to split the strongly bound electron-hole pairs (excitons), ABX3 perovskites sustain weakly bound and short-lived excitons that spontaneously break apart at room temperature to produce free charge carriers. It is, among other factors, the long charge-carrier diffusion lengths (in excess of a micron) compared to their absorption depths that make perovskites so unique for PV applications.
We have very recently initiated a research line in the context of perovskite solar cells. We are mostly interested, at short-term, in the quantum-chemical characterization of the structural, electronic and redox properties of the hole- and electron-transporting materials with potential for applications in perovskite solar cells. At long-term, we would like to tackle the study of the organic-inorganic hybrid interface to gain more insight into the fundamental electron transfer processes that occur at this interface and that is crucial for the performance of the devices.
Selected publications:
1) I. García-Benito, I. Zimmermann, J. Urieta-Mora, J. Aragó, J. Calbo, J. Perles, A. Serrano, A. Molina-Ontoria, E. Ortí, N. Martín, Md. K. Nazeeruddin Adv. Funct. Mater. 2018, DOI: 10.1002/adfm.201801734
2) I. Zimmermann, J. Urieta-Mora, P. Gratia, J. Aragó, A. Molina-Ontoria, E. Ortí, N. Martín, Md. K. Nazeeruddin Adv. Energy Mater. 2017, 6, 1601674
3) I. García-Benito, I. Zimmermann, J. Urieta-Mora, J. Aragó, A. Molina-Ontoria, E. Ortí, N. Martín, M. K. Nazeeruddin, J. Mater. Chem. A 2017, 5, 8317.
Ionic transition-metal complexes for electroluminescent devices
OLEDs are solid-state, light-emitting devices based on the use of organic polymers or low-weight small molecules as electroluminescent materials. At present, OLEDs are a real alternative to inorganic LEDs for lighting applications since highly efficient OLEDs with lifetimes exceeding 20000 hours are regularly obtained. However, the massive entrance of OLEDs in the lighting market is limited by their high cost and tedious preparation. OLEDs are multilayer structures that are prepared by evaporation techniques in high-vacuum chambers, and that need rigorous encapsulation to prevent the degradation in air due to the reactive metals (Ba, Ca, Mg) used as cathodes. An alternative to OLEDs in lighting applications are the so called light-emitting electrochemical cells (LECs). LECs present a very simple structure involving a single electroactive layer processed from solution, operate with air-stable metals (Al, Ag, Au) as cathodes, which reduces the needs for encapsulation, and therefore have a lower production cost.
Figure 1 shows the basic configuration of a LEC device, which consists of the active monolayer encapsulated between the anode and the cathode. As electroluminescent material, LECs mostly use ionic transition-metal complexes (iTMCs), such as the archetype complex [Ir(ppy)2(bpy)][PF6] (Hppy = 2-phenylpyridine, bpy = 2,2’-bipyridine). When a voltage is applied, holes are injected from the anode into the HOMO of the iTCM and electrons from the cathode into the LUMO (iTMCs are oxidized and reduced). Electrons and holes migrate in the material towards the opposite electrode and a fraction of them recombine forming iTMCs in excited state (excitons) that emit light (electroluminescence). Therefore and in contrast to OLEDs, the iTMC in LECs support the three basic processes of charge injection, charge transport and light emission, thus simplifying the structure of the device. The ionic nature of the iTMC reduces the barriers for the injection of holes and electrons, making possible the use of air-stable electrodes, and facilitates the processing of the active layer from solution using spin-coating techniques. These properties determine that electroluminescent LEC devices with simple architectures can be easily fabricated from iTCMs in solution.
Since the luminescent properties of the device are largely determined by the photophysical properties of the iTMC, an in-depth knowledge of the electronic states participating in the emission process is of great interest to design new materials and improve the device performance. The MolMatTC group has a wide experience in the characterization of the electronic triplet excited states in iTMC by means of TDDFT calculations. Our main goal is to study the electronic nature (MLCT: “metal-to-ligand charge transfer”, LC: “ligand centered”, MC: “metal centered”, etc.) and the relative energy position of the emitting triplet excited states. The external quantum efficiency (EQE) and the stability (lifetime) of the device largely depend on the electronic nature of the lower-energy excited states. MLCT emitting triplets usually lead to higher photoluminescent quantum yields than LC triplets, whereas MC triplet states facilitates the chemical degradation of the complex and the quenching of the emission.
This research line presents a markedly mutidisciplinar character. In general, we study iTMC compounds with the feedback of our collaborators; Dr. Henk J. Bolink, member of ICMol and specialist in optoelectronic devices, and with the help of our synthetic partners Prof. Constable and Prof. Housecroft (Univ. Basel).
Selected publications:
1) R. D. Costa, E. Ortí, H. J. Bolink, F. Monti, G. Accorsi, N. Armaroli, Angew. Chem. Int. Ed. 2012, 51, 8178.
2) A. Pertegás, D. Tordera, J.J. Serrano-Pérez, E. Ortí, H.J. Bolink, J. Am. Chem. Soc. 2013, 135, 18008.
3) D. Tordera, J. Frey, D. Vonlanthen, E.C. Constable, A. Pertegás, E. Ortí, H.J. Bolink, E. Baranoff, Md.K. Nazeeruddin, Adv. Energy Mater. 2013, 3, 1338.